A stable isotope of helium, 3He, was produced by the Big Bang and a remnant of the solar nebula. 3He is included in ocean island basalts, such as in Hawaiian volcanos, indicating it has been stored somewhere in the deep Earth and carried up to the surface in upwelling plumes.
New computer simulations demonstrate that even in small amounts, helium can be dissolved in liquid iron at ultrahigh-pressure conditions, suggesting that there is a reservoir in the Earth’s core.
Noble gases, including helium, neon, and argon, are characterized by high chemical inertness which causes low reactivity with other materials and high volatility. Among them, 3He, 20Ne, and 36Ar are particular isotopes which were components of the primordial solar nebula existing in space before the Earth had formed.
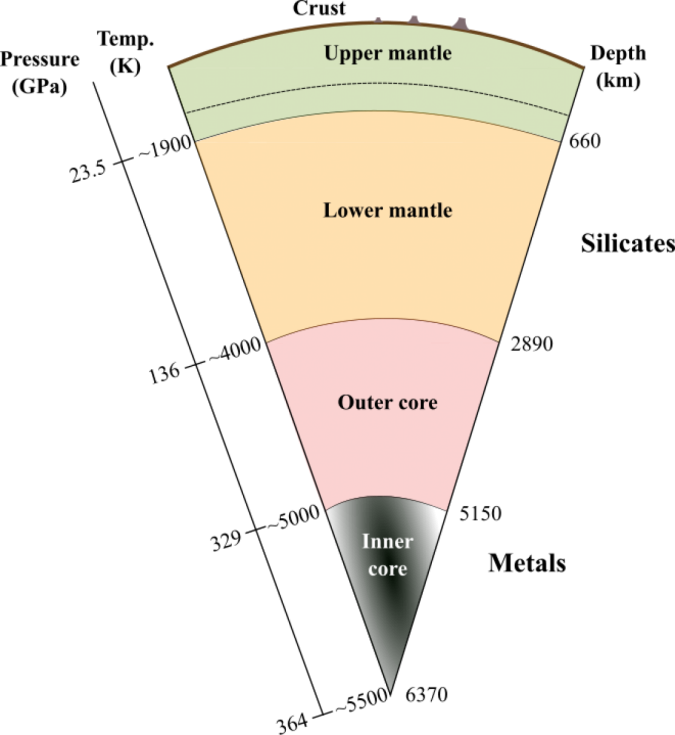
3He is also known to have been produced by the Big Bang and a substantial amount is contained in ocean island basalts, e.g., Loihi Seamount, Hawaii (e.g., Dixon et al., 2000). Such basalts are hot spot rocks which originated in the Earth’s deep interior, indicating that 3He was stored somewhere in the deep Earth.
It is surprising that such primordial helium has been confined in the Earth’s interior for 4.6 billion years, from the time of the Earth’s formation to now, even though noble gases are highly volatile. Considering the vigorous mantle convection throughout the geological time scale (e.g., Van der Hilst et al., 1997; Wang et al., 2015), it would seem unlikely that noble gases would be trapped inside the Earth so long. Although it has been suggested that the candidates for the location of the reservoir of primordial helium are the deepest mantle and the core (image 1), its location remains unclear. This is one of the biggest mysteries in deep Earth science and still under intense debate.
The outer core, composed mainly of liquid iron, is a candidate for the reservoir of primordial helium, and there is a possibility that helium is supplied from this area to the mantle. Such noble gases could be carried up to the surface with upwelling mantle plumes. This seems a reasonable scenario to explain the fact that rocks collected in the active hot spot areas, such as in Loihi Seamount and Iceland, contain high concentrations of primordial noble gases. If the outer core is the reservoir of noble gases, the necessary amounts would have to be dissolved in liquid iron under high pressure.
However, previous experimental studies reported that at relatively low pressures from 1 atm to 20 GPa, noble gases generally prefer silicates (the mantle) to metals (the core) (e.g., Bouhifd et al., 2013). The property by which a particular solute is dissolved into different coexisting solvents in different amounts is called element partitioning.
On the other hand, there exists no study so far which has investigated the property of metal/silicate partitioning of noble gases at pressures of 10 GPa to 100 GPa, corresponding to the conditions where the Earth’s proto core reacted with the magma ocean in the early stage of the Earth’s formation. Therefore, it is hard to exclude the possibility that the core is a reservoir of noble gases. If noble gases change to prefer metals with increasing pressure (a property called siderophile), more could be dissolved into the core, and it is important to clarify the partitioning properties of noble gases.
Precise experimental measurements of elements partitioning under high pressure are quite difficult, so in this study, by means of the quantum mechanical computer simulation technology called the ab initio method, the partitioning properties of helium and argon between liquid iron and molten silicate (magma) were investigated in the wide pressure range of 20 GPa to 135 GPa.
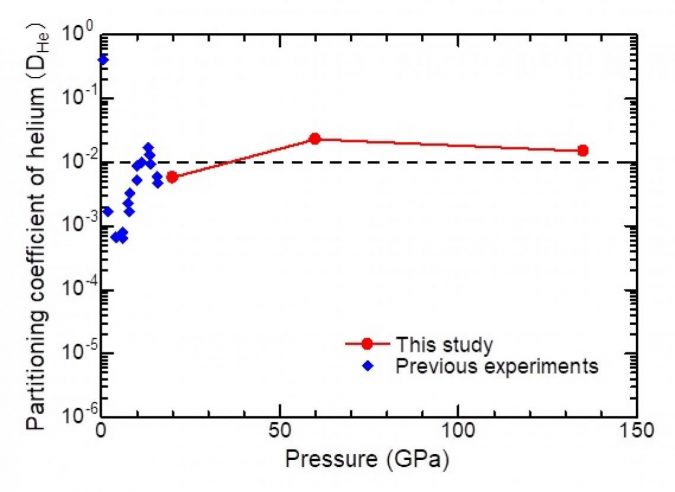
@Taku Tsuchiya, Ehime University
Computer simulations of element partitioning were conducted by calculating the reaction energies when noble gases are dissolved into liquid iron and molten silicate. By comparing these reaction energies, the relative differences in the equilibrium of the noble gas concentrations in coexisting liquid iron and molten silicate could be estimated. Based on the fundamental principle of thermodynamics, noble gases are dissolved more into a solvent with a smaller reaction energy, and thus larger differences in the reaction energies more greatly enhance the contrast in the noble gas concentrations in liquid iron and molten silicate.
Special techniques are required to compute the reaction energies of noble gases with liquids such as liquid iron and molten silicate. In this study, this was conducted by combining a method called the thermodynamic integration method, authorized by statistical mechanics, with the ab initio molecular dynamics method (Taniuchi and Tsuchiya, 2018; Xiong et al., 2018).
The calculations of the partitioning properties of noble gases between liquid iron and molten silicate obtained by these original techniques indicate for the first time in the world that noble gases remain, preferring molten silicate to liquid iron up to the core-mantle boundary pressure (135 GPa), and there is no distinct increase in their siderophility.
The amount of helium dissolved in the core in the early stage of the Earth’s formation is considered to be approximately 1/100 of the amount dissolved in the mantle (image 2). (In contrast, argon is found to become more siderophile with increasing pressure. The different high-pressure behaviors are caused by the different atomic sizes of helium and argon.) This result, showing no considerable pressure effects, suggests that the core is unsuitable as the primordial reservoir, but the estimated total amount of 3He stored in the core is, even if only 1/100, enough to explain the 3He flux measured in the present hot spots.
Even though 100 times more helium was dissolved into the magma ocean, most of it would have evaporated into the air while it solidified and only marginal amounts would be left due to its high volatility. In contrast, helium dissolved in the core during the proto core formation in the magma ocean was confined to the core after the magma ocean solidified.
It is considered that such helium has been gradually seeping into the mantle across the core-mantle boundary and ascending to the surface with upwelling plumes over a long period of time. It can be measured in the hot spot rocks even now. These results provide conclusive support showing that the 3He reservoir is at the core. This is an important insight for the location of the primordial reservoir, one of the long-standing mysteries in Geoscience.
References
Bouhifd, M. A., Jephcoat, A. P., Heber, V. S., & Kelley, S. P. (2013). Helium in Earth’s early core. Nature Geoscience, 6(11), 982–986.
Dixon, E., Honda, M., McDougall, I., Campbell, I., & Sigurdsson, I. (2000). Preservation of near solar isotopic ratios in Icelandic basalts. Earth and Planetary Science Letters, 180, 309–324.
Taniuchi, T., & Tsuchiya, T. (2018). The melting points of MgO up to 4 TPa predicted based on ab initio thermodynamic integration molecular dynamics. Journal of Physics: Condensed Matter, 30(11), 114003.
Van der Hilst, R. D., Widiyantoro, S., & Engdahl, E. R. (1997). Evidence for deep mantle circulation from global tomography. Nature, 386(6625), 578–584.
Wang, X., Tsuchiya, T., & Hase, A. (2015). Computational support for a pyrolitic lower mantle containing ferric iron. Nature Geoscience, 8(7), 556–559.
Xiong, Z., Tsuchiya, T., & Taniuchi, T. (2018). Ab initio prediction of potassium partitioning into Earth’s core. Journal of Geophysical Research: Solid Earth, 123, 6451–6458.
(Provided by Ehime University Geodynamics Research Center via AsiaResearch Sea)