The computer model, developed under the lead management of researchers at TU Graz, simulates the cyclical changes in the membrane potential of a cancer cell using the example of human lung adenocarcinoma and opens up completely new avenues in cancer research.
Digital cell models have so far focused on excitable cells such as nerve or cardiac muscle cells, allowing the simulation of electrophysiological processes not only at the cellular level, but also at the tissue and organ level. These models are already being used to support diagnosis and therapy in everyday clinical practice. The international research team led by Baumgartner focused on the specific electrophysiological properties of non-excitable cancer cells for the first time.
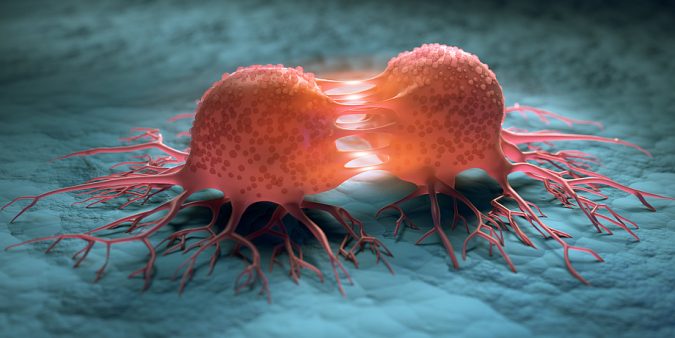
In excitable cells, an electrical stimulus triggers so-called action potentials. This leads to short-term changes in electrical potential lasting milliseconds at the cell membrane that transmit electrical information from cell to cell. Through this mechanism, neural networks communicate or the heart muscle is activated, which contracts as a result. It is known from experimental studies that non-excitable cells also exhibit characteristic fluctuations of potential at the cell membrane.
However, compared to excitable cells, the potential changes occur very slowly and over the entire cell cycle, i.e. over hours and days, and serve as a signal for the transition between the individual cell cycle phases, explains Baumgartner. Together with the deputy head of the institute, Theresa Rienmüller, and Ph.D. student Sonja Langthaler, Baumgartner was the first to pursue the idea of developing a simulation model of these mechanisms.
Pathological changes in cell membrane voltage, particularly during the cell cycle, are fundamental to cancer development and progression.
“Ion channels connect the outside to the inside of a cell. They enable the exchange of ions such as potassium, calcium or sodium and thereby regulate the membrane potential. Changes in the composition of ion channels, as well as altered functional behaviour of the same, can result in disruptions in cell division, possibly even affecting cell differentiation and thus transforming a healthy cell into a diseased (carcinogenic) cell,” said Langthaler.
For their digital cancer cell model, the team chose the example of the human lung adenocarcinoma cell line A549. The computer model simulates the rhythmic oscillation of the membrane potential during the transition between cell cycle phases and enables prediction of the changes in membrane potential that are caused by drug-induced switching on and off of selected ion channels.
“So, we get information about the effects of targeted interventions on the cancer cell,” Baumgartner adds.
The activity of certain ion channels can also drive the division of diseased cells and thus accelerate tumour growth. If ion channels are now manipulated in a targeted manner, as is the case with new, promising agents and drugs, the cell membrane voltage and thus the entire electrophysiological system can be thrown off track, so to speak.
“This could be used to arrest cancer cells at a certain phase in the cell cycle, but also to induce premature cell death (apoptosis). One could freeze cancer cells while they are growing or induce them to commit suicide. And it is precisely such mechanisms that can be simulated with the help of models,” said Baumgartner.
He and his team see the first digital cancer cell model as the beginning of more comprehensive research. In order to increase the level of detail of the model, plans for further experimental and measurement validations have been made and submitted to the Austrian Science Fund FWF for funding.